Electrical power grid control
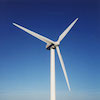
The accommodation of uncertain forecasts is one of the most pressing challenges in the control of power systems with a high penetration of intermittent renewables, such as wind and solar power. Our recent work has demonstrated the use of robust optimization with multi-stage recourse policies to provide reserves much less conservatively than with existing means. Our approach is particularly attractive for ensuring that devices such as plug-in electric vehicles, pumped hydro plants, batteries, and building HVAC can participate in reserve provision while still making guarantees on future availability of stored energy.
IfA's EPGC (Electrical Power Grid Control) activities fall into four categories:
- chevron_right Multistage recourse policies for multiperiod power system optimization under uncertainty
- chevron_right Distributed mechanisms for solving AC and "DC-approximated" optimal power flow
- chevron_right Slow coherency and coordinated wide-area control
- chevron_right Distributed control and optimization in microgrids
Multistage recourse policies for multiperiod power system optimization under uncertainty
This work makes use of recent results in robust optimization and control in order to choose optimal planned feedback rules for power system devices such as generators, energy storage units, and curtailable renewable infeeds. These feedback rules are known as reserve policies, or decision rules, and prediction error statistics and bounds to be incorporated into a multi-period look-ahead dispatch. This has been shown to have the effect of reducing the cost of uncertainty relative to more conventional reserve mechanisms.
Distributed mechanisms for solving AC and "DC-approximated" optimal power flow
This work focuses on the use of methods such as Lagrangian relaxation and the Alternating Direction Method of Multipliers to solve power flow problems, motivated by two factors:
- Privacy requirements of market mechanisms;
- A natural decomposition structure present in the problem.
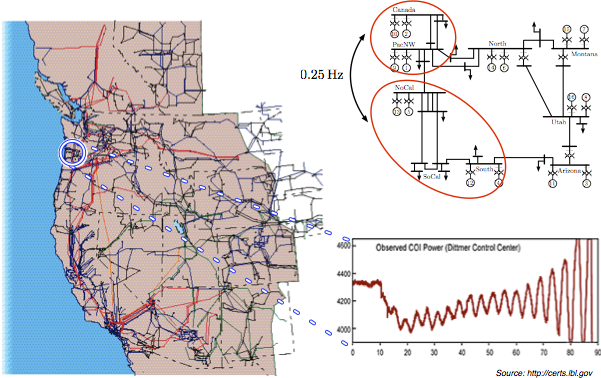
A power network is a large-scale and complex dynamical system combining both the rich dynamics of the individual subsystems as well as their non-trivial interaction through the network. In this project, we are interested in the electromechanical inter-area oscillations, which are associated with the dynamics of power transfers and involve groups of generators oscillating relative to each other. To tackle the complexity of inter-area oscillations for analysis, control design, and monitoring schemes, it is of interest to construct reduced-order models which preserve the dynamics of interest. We follow the slow coherency approach based on identifying and aggregating sparsely and densely connected areas of a network, within which all generators swing coherently. Since inter-area oscillations are typically poorly controllable by means of local control, recent efforts have been aimed at developing wide-area control strategies that involve communication of remote signals. Rather than fixing the control structure a priori, our work employs the recently-introduced paradigm of sparsity-promoting optimal control to simultaneously identify the optimal control structure and optimize the closed-loop performance.
Distributed control and optimization in microgrids
With the goal of integrating distributed renewable generation and energy storage systems, the concept of a microgrid has recently gained popularity. Microgrids are low-voltage electrical distribution networks, composed of distributed generation, storage, load, and managed autonomously from the larger transmission network. Microgrids are able to connect to a larger electric power system, but are also able to island themselves and operate independently. Modeled after the hierarchical control architecture of power transmission systems, a layering of primary, secondary, and tertiary control has become the standard operation paradigm for microgrids. Despite this superficial similarity, the control objectives in microgrids across these three layers are varied and ambitious, and they must be achieved while allowing for robust plug-and-play operation and maximal flexibility, without hierarchical decision making and time-scale separations. Our work focuses scalable, robust, and plug'n'play control and optimization strategies for the problems of synchronization and load sharing, voltage stabilization, secondary regulation and economic dispatch, as well as their experimental validation in microgrids.